Instant CEMTRO Cell (ICC), high density autologous chondrocytes implantation
Instant CEMTRO Cell (ICC), implante de condrocitos autólogos de alta densidad
Resumen:
La medicina regenerativa es una nueva disciplina de la medicina, con un futuro muy prometedor para el tratamiento de muchas dolencias que aquejan al ser humano. Para muchas enfermedades todavía está en fase de experimentación. Uno de los campos en los que más ha avanzado es en el tratamiento del cartílago, desde la utilización de cultivos de condrocitos autólogos en medio líquido, al empleo de biomateriales como la membrana de colágeno I/III y, finalmente, al aumento de dosis celular en la técnica ICC. Por ello, en las lesiones de cartílago de grado III/IV de Outerbridge de rodilla, tobillo y ahora ya en cadera, podemos usar la técnica de implante de condrocitos en membrana (ICC), consiguiendo la regeneración del tejido cartilaginoso.
Se trata de medicina regenerativa, pues se forma un tejido cartilaginoso de naturaleza hialina con la misma estructura y función que el cartílago articular sano. Además, los pacientes presentan una recuperación funcional excelente, consiguiendo la vuelta al deporte en muchas ocasiones.
Abstract:
Regenerative medicine is a novel medical discipline with very promising perspectives for the treatment of numerous human disease conditions. In many such conditions it is still in the experimental phase. One of the fields in which the greatest advances have been made is cartilage treatment, ranging from the use of autologous chondrocyte cultures in a liquid medium to the utilization of biomaterials such as type I/III collagen membranes, and lastly cell dose augmentation with the Instant CEMTRO Cell (ICC) implantation of chondrocytes in membrane technique. In this regard, in Outerbridge grade III/IV cartilage lesions of the knee, ankle and now also the hip, the ICC technique can be used to regenerate the cartilage tissue.
This is a regenerative medical technique, since hyaline cartilage tissue is formed with the same structure and function as the healthy joint cartilage. Furthermore, patients experience excellent functional recovery and are often able to return to sports activities.
Introduction
Although cartilage damage is most often of idiopathic or degenerative origin, joint cartilage injuries are also frequently caused by traumatisms (Figure 1A), i.e., joint trauma, followed by osteochondritis dissecans (OCD)(1,2) (Figure 1B).
Other factors that also result in joint degenerative changes comprise articular instabilities secondary to failures that cause meniscal and cartilage damage due to shearing forces or misalignments of the knee joint axis. As a consequence of overload or compression mechanisms, these situations induce progressive joint damage that may prove asymmetrical by affecting the internal, external or femoropatellar compartment on an isolated basis. On progressing, these disorders ultimately result in tricompartmental alterations with generalized osteoarthrosis of the knee, in which the treatment options are limited to joint replacement surgery or joint fixation(1,2).
The chondral defects may be partial or complete, depending on whether the full thickness of the cartilage is affected, with involvement of the subchondral bone. In this case a repair mechanism is activated that does not produce normal cartilage but rather repair tissue lacking the biomechanical characteristics of healthy hyaline cartilage, due to a decrease in type II collagen, which is replaced by type I collagen. In addition, the chondrocytes are replaced by fibroblasts, which are unable to produce an extracellular matrix with the adequate concentration of type II collagen(3,4) (Figure 2).
Classification of cartilage lesions
Although there are a number of classifications for assessing the degree of joint cartilage damage, the most widely used option is the classification of Outerbridge (1961), which defines four grades(5,6) (Figure 3):
• Grade I: softening or edema of the cartilage surface.
• Grade II: fissures or fragmentation affecting a surface of less than 1.25 cm in diameter.
• Grade III: fissures or fragmentation affecting a surface of over 1.25 cm in diameter.
• Grade IV: erosion of the cartilage extending to the subchondral bone.
Figure 4 shows arthroscopic images of lesions corresponding to grade II (Figure 4A), grade III (Figure 4B) and grade IV (Figure 4C).
reaca.28171.fs1909039en-figure5.png
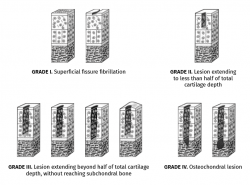
Figure 5. Schematic representation of the classification of joint cartilage lesions of the International Cartilage Repair Society (ICRS). Figure adapted from the chondral lesion assessment form. ICRS Cartilage Injury valuation Package; 2000. Retrieved from: https://cartilage.org/content/uploads/2014/10/ICRS_evaluation.pdf.
The classification of the International Cartilage Repair Society (ICRS) also establishes four grades, but moreover contemplates sub-grades(7) (Figure 5):
• Grade 0: normal.
• Grade 1: near normal.
– A: superficial fibrillation or softening of the cartilage.
– B: superficial fissures or lacerations.
• Grade 2: abnormal, lesions corresponding to < 50% of cartilage depth.
• Grade 3: severely abnormal.
– A: lesions corresponding to > 50% of cartilage depth, without reaching the calcified layer.
– B: lesions corresponding to > 50% of cartilage depth, reaching the calcified layer.
– C: complete defect, with involvement of the subchondral bone plate.
– D: blistering appears.
• Grade 4: severely abnormal.
– A: osteochondral lesions extending to the subchondral bone plate.
– B: deep defects affecting the trabecular bone.
The classification of cartilage lesions allows definition of the lesion and may contribute to establish a treatment protocol. Although the classifications are not one hundred percent reliable, there appears to be agreement on their use - especially those classifications based on direct visualization(8).
Improvements in knowledge of cartilage disease and in radiodiagnostic imaging techniques will allow the definition of reliable classifications, before arthroscopy becomes necessary - thereby allowing us to treat the cartilage from the first lesion grades.
Regenerative medicine
Regenerative medicine - a term introduced by William Haseltine in 1999 - has been one of the major scientific developments of recent years, assisted by the great biological advances made following decoding of the human genome and epigenome(9). The genome contains the instructions referred to the definition of our cells and how they are going to function, while the epigenome provides the instructions that regulate gene activation and cell functions. Regenerative medicine is a branch of translational research focused on the replacement of damaged cells, tissues or organs, with a view to restoring their structure and function. The main objective of this new field in medicine is to restore functions or organs that have been lost, and this could be achieved from different perspectives(10,11).
Cells, tissues, organs and systems
During embryonic development, a cell (the zygote) multiplies to give rise to more cells. These in turn divide and differentiate according to the functions they will carry out later on, and finally give rise to the whole individual(12). In the course of this process, the cells organize into structures of increasing complexity. The cell is the basic morphological and functional unit of life. In multicellular organisms, tissues constitute the first level of cell organization. Tissues are composed of different cells that share a common embryonic origin and act together to perform a common function. There are four main types of body tissues, according to their structure and function: epithelial tissue, connective tissue, muscle tissue and nervous tissue.
Normal tissue essentially consists of three components(12):
• Extracellular matrix, or the combination of materials of a complex biochemical nature that surround and embed the cells, providing support and acting as a framework for the tissues. The extracellular matrix interconnects the cells within a tissue, serving as a cohesive and integrating medium that is essential for ensuring that the cells work in a cooperative manner.
• Chemical mediators, or molecules that act via receptors as communication signals between the external medium and the cells that conform the tissue, with the capacity to modulate cell responses.
• Cells, which are the living functional units of the tissues and are able to divide and synthesize molecules to interact with the medium.
The next level of organization is represented by organs, which are composed of two or more different types of tissues with the purpose of performing a concrete function. Finally, tissues and organs group to form systems that carry out certain physiological functions such as for example breathing or digestion(12).
Thus, what we do is create a tissue from the cells it is made of. These are the cells that produce the extracellular matrix.
Regeneration versus repair
All tissues undergo constant renewal, with cell death and tissue renovation. This is a constant process, and its duration, and particularly the extent of renewal, depend on the type of tissue involved. On the other hand, this process is not perfect, and its efficiency decreases with aging - a fact that sometimes may give rise to diseases and is ultimately related to the aging process. The restoration of a damaged tissue or organ may occur via two independent pathways: regeneration (when tissue identical to the damaged tissue is formed) or repair (when tissue of similar but not identical characteristics is formed, and which in some cases may simulate the functions of the original tissue - as with scarring following a skin wound - though not so in other cases)(13).
The cell as a medication
Regenerative medicine combines cell biology techniques with other disciplines for the production of new organs and tissues(11). This new approach to organ and tissue substitution was introduced in the late twentieth and early twenty-first century, with the cell as the element playing the true leading role(14). The trajectory started in the nineteenth century when scientists such as Roux and Ringer developed techniques for the in vitro culture of cells, in which the latter were removed from their natural environment. Over the remaining years of that century, and particularly throughout the twentieth century, these techniques were gradually improved upon, and a great variety of technological advances were introduced, such as laminar flow chambers, cell incubators, bioreactors or white rooms, among others, that have allowed cell cultures to be performed on a routine and (particularly) safe basis - avoiding contamination by microorganisms as the main problem in such processes. All this currently allows us to speak of cells as a medication, since they can be cultured under the same conditions and in environments as sterile and controlled as those found in relation to medicines(14).
However, in order for cell application as a medication to be safe, it is necessary for regulatory agencies to intervene in the corresponding utilization and of course manufacturing processes. In 2007 a European Directive was introduced, defining for the first time these new medications based on the use of cells. The Directive encompassed them within a new category of drugs called advanced therapy medicinal products, and which include three types of medications(15). The term "advanced therapy" is curious, though the authorities underscore that it is not intended to downplay the importance of traditional medicines. Rather, the term refers to the fact that these are medications in which the main component is alive, and that it is mainly obtained through the culture of cells from higher organisms and following a long research process. Within this category of advanced therapy medicinal products, three types can be distinguished: cell therapy, tissue engineering and gene therapy(15) (Table 1).
The mentioned European Directive further establishes the way to culture these cells which will play a therapeutic role: since they are medicines, the cells must be cultured in a "clean" or sterile environment that is the same as that used to manufacture other medications(15,16). In order for these cells to reach the category of medicinal products, the Directive moreover requires their "manufacture" or more exactly their culture process to adhere to a series of quality standards referred to as Good Manufacturing Practices (GMP)(16). Only in this way can cells constituting an advanced therapy medicinal product guarantee the required therapeutic quality for use in humans(16).
Consolidated therapies
In the year 2014, the Spanish Official State Bulletin (Boletín Oficial del Estado) published a Royal Decree regulating the non-industrial production of medicinal products, with mention for the first time of concepts such as exclusion clauses and consolidated therapies. The special nature of advanced therapy medicinal products was recognized for the first time, and in particular a fundamental difference was underscored with respect to traditional medicinal products in relation to production. In effect, while traditional medications are manufactured in industrial plants, medications based on cells are usually produced in white rooms located in facilities with GMP certification, and not on an industrial basis - particularly in the case of cell-based medications of an autologous nature(17). The mentioned Royal Decree establishes that all cell therapies, except those recognized as consolidated therapies, must go through all the clinical research phases until receiving approval as medicinal products. Consolidated therapies are treatments whose efficacy and safety have been demonstrated over years of use in humans, before cell therapies became classified as medicinal products, with abundant supporting scientific evidence. The Decree acknowledges three consolidated therapies: keratinocyte culture for the treatment of skin burns; corneal limbal cell culture for the treatment of corneal ulcers; and autologous chondrocyte culture for the treatment of focal joint cartilage damage.
Implantation of autologous chondrocytes in suspension
Autologous chondrocyte implantation (ACI) is based on the use of autologous chondrocytes in suspension(18). The chondrocytes are isolated from a cartilage biopsy sample taken from a non-weight bearing zone, and are then cultured and implanted in the damaged cartilage region, where they contribute to its regeneration(18). This method allows the successful repair of joint defects with a surface of less than 10 cm2, and with a depth that may even reach the subchondral bone. In this technique, the chondrocytes are grafted into the chondral or osteochondral defect under a periosteal patch obtained from the tibia, and which is sutured to the cartilage(18,19). Cartilage regeneration occurs in most cases (almost 80% of all treated cases), with a considerable decrease in pain and inflammation, and substantial improvement of joint mobility(20,21).
Autologous chondrocyte implantation in a liquid medium is a technique that affords very good results, though it does pose some problems(22). Firstly, implantation of the cells during the surgical procedure is complicated, since they are contained in a liquid medium, with handling difficulties, and cannot be applied via arthroscopy. Furthermore, the implant zone must be covered with periosteum, which poses two problems. Firstly, the harvesting of periosteum requires greater surgery in that the tibia must be accessed, and this increases the morbidity associated to the technique. Secondly, since this is a living tissue, hypertrophic growth may result, with posterior delamination of the implant(23).
This technique began to be used in Spain in 1996 for the treatment of cartilage lesions of the knee and ankle. The cultures were first made in Sweden and posteriorly in the United States, and were shipped to Spain under conditions optimum for survival of the cells. Cell culture was performed under two indications:
• When arthroscopic evaluation considered ACI to be appropriate: immediate indication.
• When the patient continued to experience problems following an arthroscopic treatment (microfractures, perforations), and ACI was then prescribed as rescue therapy: late indication.
Accordingly, between 1996-2001, the cumulative series of the Clínica CEMTRO comprised a total of 223 biopsies, with ACI therapy performed in 152 of them. A total of 152 patients were operated upon, with a mean age of 30 years (range: 14-56 years), 125 males and 27 females (Figure 6A). With regard to the professions of these patients, 101 were athletes while the rest had sedentary occupations.
The number of defects treated and their location are described in Figures 6B and 6C. The mean defect size was 6.1 cm (range: 1.5-13.5 cm).
With regard to the ankle lesions, the location was in the astragalus in all 6 cases: the upper-internal quadrant in four cases and the upper-external quadrant in two.
One of these cases was the first case reported in the world of the application of the technique to ankle lesions. A malleolar osteotomy (external or internal) was performed in all 6 cases, followed by fixation with a screw. Immobilization was not used, and the patients were able to mobilize the ankle immediately. Weight bearing was avoided during 8-10 weeks.
Overall, the outcomes have been very good, with follow-up extending from 1996 (when the first case was implanted) and 2001 (when the next technical modality was adopted) to date (17-22 years of follow-up). Seventy-five percent of the patients have been able to lead a normal life, including elite sports activities in some cases. Thus, the ACI technique has yielded very satisfactory results.
The use of carriers in cell therapy. Implantation of autologous chondrocytes on collagen membranes
The application of ACI on a carrier or scaffold has been a great advance in the treatment of joint cartilage lesions. One of the most commonly used techniques worldwide is the matrix-assisted autologous chondrocyte implantation (MACI), in which a type I/III collagen membrane of porcine origin is used as biomaterial(24,25,26,27,28). This technique is based on a modification of the ACI procedure, involving the use of a collagen membrane that acts as a biological support in which the chondrocytes are integrated after culture(24). This technical variant is employed to cover the cartilage defect with the mentioned membrane instead of using periosteum (as a patch). An advantage of MACI is that it can be performed with arthroscopic techniques and, since no periosteum is required, surgery is simpler(29). Since in this case implantation was made with a membrane, in our centre we designed special instruments to facilitate placement of the cultured cells (Figure 7).
The inconvenience of this technique is that the placed cell concentration is low: 1,000,000 cells per cm2.
The results obtained with this variation of the technique have been very good, and by using the arthroscopic approach it is still possible to achieve practically complete regeneration of the joint surface in a high percentage of cases through the formation of hyaline cartilage rich in type II collagen(25,28).
In the Clínica CEMTRO, the MACI technique was used in 174 patients during the period 2001-2010. The results of the 50 patients treated with MACI in our centre were published in 2015(30).
The mean patient age was 26 years (range: 14-48 years). In 42 cases the lesion was located in the knee and in 8 cases in the ankle. The preoperative diagnosis was osteochondritis dissecans (OCD) in 38 cases. Of the 50 patients, only 9 had not undergone any previous surgery. In 42 cases previous surgery had been performed, mainly in the form of arthroscopies or surgeries for the treatment of OCD (Figure 8).
The defect size was less than 2 cm2 in 35 cases. Implant success was assessed on the basis of pain variation before and after surgery using the visual analogue scale (VAS). This scale scored pain as follows:
• 0-3: no pain or discomfort.
• 3-6: pain allowing normal activities of daily living, but not sports.
• 6-10: pain limiting normal activities of daily living.
As can be seen in Table 2, a significant decrease was recorded in the percentage of patients with pain before and after surgery (p < 0.0001; Pearson chi-square test).
Mobility after surgery was complete in all but four cases, which presented limitation over the last degrees of flexion. Of the 50 patients, 32 (64%) were able to resume their sports activities while 21 (36%) did not return to the sports activities they had before the injury - though in a number of cases some type of sports proved possible, such as swimming or bicycling. Percentage improvement among the patients that had undergone previous surgery was 69% versus 89% among those without previous surgery (Fisher exact test; p = 0.1442). Although the difference was not statistically significant, the outcomes tended to be better among those individuals that had not undergone previous surgery.
In 5 cases a biopsy of the regenerated tissue could be obtained two years after implantation. The histological study revealed chondrocytes surrounded by a cartilaginous matrix, distributed in clusters, and with a columnar distribution in some areas (Figure 9).
Instant CEMTRO Cell: high density autologous chondrocytes implantation
In 2008, the Clínica CEMTRO, together with Laboratorio Amplicel, started the development of a proprietary ACI method based on membranes for the treatment of cartilage lesions(31). This technique also uses the same biomaterial as in MACI, i.e., a type I/III collagen membrane of porcine origin. The membrane has a permeable rough surface where the cells are deposited, followed by placement in contact with the lesion, and a non-permeable smooth surface that prevents the cells from "escaping" from the membrane into the joint cavity (Figure 10).
The properties of the type I/III collagen membrane that make it suitable as a biomaterial for chondrocyte implantation are:
• The carrier is mechanically stable.
• It is biocompatible.
• The membrane is resistant and can be applied to the defect using biological fibrin glue; suturing is also possible.
• The membrane is biodegradable and is reabsorbed within 3-6 months.
This membrane, which is also used in the MACI implant, does not require the use of periosteum, and the procedure can be carried out via arthroscopy - suturing the implant to the bone tissue and sealing with fibrin glue (Figure 11).
During preparation of the method, we considered it interesting to use a modification of the MACI technique, for although the results obtained were good, the newly formed cartilage was softer and presented less cellularity than the original tissue. In the MACI technique, the cells are cultured until reaching a count of 20-30 million (approximately 6 weeks of culture), which is the amount applied in the collagen membrane measuring 20 cm2 in size; the cell density therefore is one million cells per cm2 of damaged zone. Thus, according to this technique, in the case of a lesion measuring 2 × 2 cm2 in size, we actually would be implanting four million cells, with a "waste" of 16 million (Figure 12).
Our modification involves elevating the cell density in the membrane with the aim of applying a greater number of cells. The results obtained from our tests to optimize cell density on the membrane showed that the cell dosage could be increased 5-fold(31).
In this procedure, during implant surgery we measure the lesion and trim the membrane according to the shape and size of the former. Once the membrane has been trimmed, the entire autologous chondrocyte culture is applied onto it (5 million chondrocytes per cm2 of membrane), and after the required waiting time (10 minutes) to ensure absorption, it is implanted in the patient. This improves the yield of the technique. We call this modification Instant CEMTRO Cell (ICC) or high density autologous chondrocyte implantation (HD-ACI). Figure 13 provides a schematic representation of the ICC technique and the main difference with respect to the MACI procedure.
Instant CEMTRO Cell autologous chondrocyte implantation procedure
Our proposal to increase the cell dosage (5 million cells/cm2 of lesion) affords notorious improvement of the results. Figure 14 provides a schematic representation of the ICC procedure.
Production process
Figure 15 schematically presents the autologous chondrocyte culture process under GMP conditions. Good Manufacturing Practices (GMP) are known as Total Quality Management standards, and ensure that all the variables intervening in production are fully controlled - ensuring the quality of all the cultures produced.
The following analytical controls are carried out during the cell production process:
a) Sterility test: this is performed in four phases of the process.
b) Mycoplasma test: Mycoplasma is an intracellular bacterium that can contaminate cell cultures and is difficult to detect, since it does not cause turbidity of the culture medium. This test is performed in three phases of the production process.
c) Bacterial endotoxins test: this is performed at a single point of the process.
d) Cell phenotyping: one of the problems that can arise during the cell production process is that the cells may undergo dedifferentiation over the successive passages (subcultures).
In the case of chondrocytes, phenotyping is made using real time polymerase chain reaction (PCR) technology to determine the expression of type II collagen and aggrecan (genes characteristic of hyaline cartilage).
Clinical application of the Instant CEMTRO Cell high density chondrocyte implantation procedure
In the period between 2010 and 2018, a total of 245 patients underwent ICC in the Clínica CEMTRO and other hospitals (Table 3) for the treatment of chondral defects of the knee, ankle and hip.
Careful patient selection is important in order to secure good outcomes with ICC therapy. The ICC technique is indicated for the repair of Outerbridge grade III-IV or ICRS grade III-IV symptomatic chondral and osteochondral lesions of the joints. More than one lesion of the same joint can be treated, according to the criterion of the traumatologist. The lesions may be caused by acute trauma (e.g., falls), repetitive trauma (as in incorrect knee support during a long period of time), or OCD. The ICC technique is indicated for all synovial joints (knee, ankle or hip), though it is most commonly used in application to the knee and ankle. If the joint presents some other meniscal lesion, luxation, instability or axial alteration, it must be treated independently and before the cartilage implant, or in the same surgical procedure.
reaca.28171.fs1909039en-figure16.png
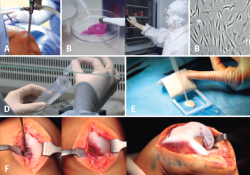
Figure 16. A: biopsy obtainment; B: cartilage sample (amount 3-4 g with size of rice grains); C: cell culture in white room under GMP conditions; D: after 6-8 weeks the adequate number of cells for implantation are obtained (20-30 million, depending on the size and number of lesions); E: cell seeding in the membrane (biomaterial) in the operating room, at a density of 5 million cells/cm2; F: implantation of the membrane with cells. Membrane suturing.
The general ICC implantation procedure is as follows (Figures 16 and 17):
• Arthroscopy is used to obtain a biopsy from a non-weight bearing zone.
• The biopsy is sent to the cell therapy laboratory for cell culture.
• After 4-6 weeks the required number of cells are obtained, depending on the number of lesions and their size (5 million cells/cm2 of lesion).
• During cell implantation, the membrane is trimmed according to the shape and size of the lesion, and the entire cell culture (at least 20 million cells) is seeded in the membrane.
• Once the cells have been absorbed by the membrane, the latter is implanted at the lesion site.
Patients with chondral defects of the knee: results of the first 50 cases with two years of follow-up
The results obtained with the first 50 patients subjected to treatment of the knee with ICC and involving two years of follow-up have recently been published(32). The studied patients presented a median age of 35 years (range: 18-49 years). Of these, 70% were males, and in 54% of the cases the chondral defect was located in the right knee. Most of them (72%) had undergone a previous surgery. In 66% of the cases the defect was of a chondral nature, in 20% the lesion was osteochondral, and in 14% the diagnosis was OCD (Figure 18). In the patients with previous surgery, the latter was carried out due to cartilage problems in 22 cases - the most frequent condition being microfractures (in 50% of the cases with previous cartilage surgeries).
The efficacy and safety of the technique were assessed based on the following variables:
1. Presence of pain.
2. Effusion.
3. Mobility range (flexion and extension).
4. Subjective perception of knee condition based on the International Knee Documentation Committee (IKDC) scale.
Imaging studies (radiographs and magnetic resonance imaging [MRI]) were made before and during follow-up to assess implant outcome (at 6, 12 and 24 months).
As can be seen in Table 3, both pain and effusion improved significantly over follow-up versus the patient baseline condition. With regard to mobility, flexion also improved significantly with respect to the baseline situation.
The IKDC questionnaire evaluates the subjective perception of the patient regarding the functional condition of the knee(33). The score ranges from 0-100, where higher scores correspond to improved knee condition. Statistically significant improvement of the IKDC scores was recorded. The patients improved significantly one and two years after treatment.
The improvement in mean IKDC score versus the baseline visit was 26.3 points (95% confidence interval [95%CI] of the mean: 18.2-34.4 points) at 12 months and 31.0 points (95%CI of the mean: 22.9-39.0 points) at 24 months. The minimal clinically important difference (MCID)(34) indicates the minimum difference required of the value of a given parameter in a period of time in order for the patient to perceive it as representing real improvement of that parameter. In the case of the IKDC score, the reported MCID at 12 months is 16.7 points. This means that in our case, where the scores were greater than the MCID (26.3 at 12 months and 31 at 24 months), our patients perceived the difference as representing real clinical improvement.
Patients with osteochondral defects of the ankle: results of the first patients with two years of follow-up
A study was made of the first 52 patients with osteochondral defects of the ankle treated with the ICC technique - most of them (34 patients) in the Clínica CEMTRO(35). One of the particularities of the chondrocyte implant procedure in the ankle is that in most cases an osteotomy is first required in order to access the lesion. Although in some patients the defect was more superficial, in the majority of cases (up to 96%) the defect was of an osteochondral nature. In 35% of the patients the lesion was over 4 mm in depth, and a cancellous bone graft was required, obtained from the osteotomy itself, above the subchondral bone, with placement of the membrane with the cells over this graft.
The short/middle term outcomes of the treated patients were very satisfactory. Both pain, evaluated by means of the VAS, and ankle function assessed by the American Orthopedic Foot and Ankle Society (AOFAS) score(36), improved significantly one year after treatment, and this improvement persisted at least one year later. The percentage of patients with good or excellent outcomes after 12 months was 75%, and this figure further increased to 79.2% at 24 months post-treatment.
The clinical results were correlated to the radiological findings, revealing correct graft integration and regeneration of the cartilage (Figure 19).
As commented above, an osteotomy is needed to access the joint, followed by reduction with osteosynthesis material during implant surgery. During withdrawal of the material, a biopsy of the newly formed tissue sometimes can be obtained. In these cases, the histological study of the biopsies of these patients confirmed the hyaline nature of the tissue, and the site of the defect was seen to be covered with newly formed tissue of characteristics similar to those of hyaline cartilage with type II collagen, in correspondence with the clinical results of the patients (Figure 20).
Figuras
Figure 2. Arthroscopic view of the repair tissue in two osteochondral lesions of the internal femoral condyle.
Figure 4. Arthroscopic views of the Outerbridge chondral lesion grades. A: grade I lesion; B: grade II lesion; C: grade III lesion.
Figure 5. Schematic representation of the classification of joint cartilage lesions of the International Cartilage Repair Society (ICRS). Figure adapted from the chondral lesion assessment form. ICRS Cartilage Injury valuation Package; 2000. Retrieved from: https://cartilage.org/content/uploads/2014/10/ICRS_evaluation.pdf.
Figure 6. Gender distribution of the patients treated with autologous chondrocyte implants (A), number of chondral defects treated (B) and their location (C).
Figure 8. Number of previous surgeries among the patients subjected to the matrix-assisted autologous chondrocyte implantation (MACI) treatment.
Figure 9. Regenerated cartilage tissue following matrix-assisted autologous chondrocyte implantation (MACI). Note the limited cellularity (A) with the chondrocytes forming clusters (B).
Figure 10. Chondro-Gide® membrane (Geistlich) used for Instant CEMTRO Cell (ICC) chondrocyte implantation.
Figure 13. Schematic representation of Instant CEMTRO Cell (ICC) autologous chondrocyte implantation.
Figure 14. Schematic representation of the Instant CEMTRO Cell (ICC) chondrocyte implantation procedure.
Figure 15. Schematic representation of the autologous chondrocyte production process (cell culture) under Good Manufacturing Practice (GMP) conditions.
Figure 16. A: biopsy obtainment; B: cartilage sample (amount 3-4 g with size of rice grains); C: cell culture in white room under GMP conditions; D: after 6-8 weeks the adequate number of cells for implantation are obtained (20-30 million, depending on the size and number of lesions); E: cell seeding in the membrane (biomaterial) in the operating room, at a density of 5 million cells/cm2; F: implantation of the membrane with cells. Membrane suturing.
Figure 18. Photograph and arthroscopic view of an example of chondral lesion in the femoral condyle due to osteochondritis dissecans.
Figure 19. Cartilage regeneration in the ankle joint after Instant CEMTRO Cell (ICC) treatment. The radiological view shows the appearance of the lesion before and after treatment. The magnetic resonance imaging view 6 months after implantation shows the start of joint cartilage regeneration.
Figure 20. Histological study of a representative case stained with hematoxylin-eosin (A), showing chondrocytes with a lacunar distribution or forming pairs, within a polysaccharide-rich matrix, as evidenced by Alcian blue staining (B).
Tablas
Table 2. Number of Instant CEMTRO Cell (ICC) implants performed since 2010. Affected joints, number of lesions and hospital centres that have performed this technique
Información del artículo
Cita bibliográfica
Autores
Isabel Guillén Vicente
Unidad de Tobillo y Pie. Clínica CEMTRO. Madrid
Unidad de Cartílago. Clínica CEMTRO. Madrid
Unidad de Rodilla. Clínica CEMTRO. Madrid
Marta Guillén Vicente
Sala de Cultivos Celulares. Amplicel
Lucía Aboli Martínez
Clínica CEMTRO. Madrid
Juan Manuel López Alcorocho
Unidad de Investigación Biomédica. Clínica CEMTRO. Madrid. España
Sala de Cultivos Celulares. Amplicel
María Dolores Pérez Pérez
Unidad de Tobillo y Pie. Clínica CEMTRO. Madrid
Medicina del Deporte. Clínica CEMTRO. Madrid
Ramón Navarro Mont
Unidad de Pie y Tobillo. Clínica CEMTRO. Madrid
Ana Belén Abenoja Lobo
Clínica CEMTRO. Madrid
Elena Rodríguez Íñigo
Clínica CEMTRO. Madrid
Pedro Guillén García
Unidad de pie y tobillo. Clínica CEMTRO. Madrid
Servicio de Traumatología. Clínica CEMTRO. Madrid
Unidad de Investigación Biomédica. Clínica CEMTRO. Madrid. España
Ethical responsibilities
Conflicts of interest. The authors declare that they have no conflicts of interest.
Financial support. This study has received no financial support.
Protection of people and animals. The authors declare that the procedures carried out abided with the ethical standards of the responsible human experimentation committee and in accordance with the World Medical Association and the Declaration of Helsinki.
Data confidentiality. The authors declare that the protocols of their work centre referred to the publication of patient information have been followed.
Right to privacy and informed consent. The authors declare that no patient data appear in this article.
Referencias bibliográficas
-
1Hjelle K, Solheim E, Strand T, Muri R, Brittberg M. Articular cartilage defects in 1,000 knee arthroscopies. Arthroscopy. 2002;18:730-4.
-
2Widuchowski W, Widuchowski J, Trzaska T. Articular cartilage defects: study of 25,124 knee arthroscopies. Knee. 2007;14:177-82.
-
3Buckwalter JA, Mow VC, Ratcliffe A. Restoration of injured or degenerated articular cartilage. J Am Acad Orthop Surg. 1994;2:192-201.
-
4Nehrer S, Spector M, Minas T. Histologic analysis of tissue after failed cartilage repair procedures. Clin Orthop Relat Res. 1999;(365):149-62.
-
5Outerbridge RE. The etiology of chondromalacia patellae. J Bone Joint Surg Br. 1961;43-B:752-7.
-
6Slattery C, Kweon CY. Classifications in Brief: Outerbridge Classification of Chondral Lesions. Clin Orthop Relat Res. 2018;476:2101-4.
-
7Brittberg M, Winalski CS. Evaluation of cartilage injuries and repair. J Bone Joint Surg Am. 2003;85-A Suppl 2:58-69.
-
8Dwyer T, Martin CR, Kendra R, Sermer C, Chahal J, Ogilvie-Harris D, et al. Reliability and Validity of the Arthroscopic International Cartilage Repair Society Classification System: Correlation With Histological Assessment of Depth. Arthroscopy. 2017;33:1219-24.
-
9Haseltine WA. Interview: commercial translation of cell-based therapies and regenerative medicine: learning by experience. Interview by Emily Culme-Seymour. Regen Med. 2011;6:431-5.
-
10Mason C, Dunnill P. A brief definition of regenerative medicine. Regen Med. 2008;3:1-5.
-
11Daar AS, Greenwood HL. A proposed definition of regenerative medicine. J Tissue Eng Regen Med. 2007;1:179-84.
-
12Stevens A, Lowe JS. Histología Humana. Madrid: Editorial Elsevier; 2006.
-
13Purnell BA, Hines PJ. Repair and Regeneration. Science. 2017;356:1020-1.
-
14De Bari C, Dell'accio F. Cell therapy: a challenge in modern medicine. Biomed Mater Eng. 2008;18:S11-7.
-
15Närhi MO, Nordström K. Regulation of cell-based therapeutic products intended for human applications in the EU. Regen Med. 2014;9:327-51.
-
16Alici E, Blomberg P. GMP facilities for manufacturing of advanced therapy medicinal products for clinical trials: an overview for clinical researchers. Curr Gene Ther. 2010;10:508-15.
-
17Ivaskiene T, Mauricas M, Ivaska J. Hospital Exemption for Advanced Therapy Medicinal Products: Issue in Application in the European Union Member States. Curr Stem Cell Res Ther. 2017;12:45-51.
-
18Brittberg M, Lindahl A, Nilsson A, Ohlsson C, Isaksson O, Peterson L. Treatment of deep cartilage defects in the knee with autologous chondrocyte transplantation. N Engl J Med. 1994;331(14):889-95.
-
19DiBartola AC, Everhart JS, Magnussen RA, Carey JL, Brophy RH, Schmitt LC, et al. Correlation between histological outcome and surgical cartilage repair technique in the knee: a meta-analysis. Knee. 2016;23(3):344-9.
-
20Brittberg M. Autologous chondrocyte implantation-technique and long term follow-up. Injury. 2008;39:S40-S49.
-
21Peterson L, Minas T, Brittberg M, Nilsson A, Sjögren-Jansson E, Lindahl A. Two- to 9-year outcome after autologous chondrocyte transplantation of the knee. Clin Orthop Relat Res. 2000;374:212-34.
-
22Dewan AK, Gibson MA, Elisseeff JH, Trice ME. Evolution of autologous chondrocyte repair and comparison to other cartilage repair techniques. Biomed Res Int. 2014;2014:272481.
-
23Kreuz PC, Steinwachs M, Erggelet C, Krause SJ, Ossendorf C, Maier D, et al. Classification of graft hypertrophy after autologous chondrocyte implantation of full-thickness chondral defects. Osteoarthritis Cartilage. 2007;15(12):1339-47.
-
24Bartlett W, Gooding CR, Carrington RW, Skinner JA, Briggs TW, Bentley G. Autologous chondrocyte implantation at the knee using a bilayer collagen membrane with bone graft. A preliminary report. J Bone Joint Surg Br. 2005;87:330-2.
-
25Bartlett W, Skinner JA, Gooding CR, Carrington RW, Flanagan AM, Briggs TW, Bentley G. Autologous chondrocyte implantation versus matrix-induced autologous chondrocyte implantation for osteochondral defects of the knee: a prospective, randomised study. J Bone Joint Surg Br. 2005;87(5):640-5.
-
26Erickson B, Fillingham Y, Hellman M, Parekh SG, Gross CE. Surgical management of large talar osteochondral defects using autologous chondrocyte implantation. Foot Ankle Surg. 2018;24:131-6.
-
27Whittaker JP, Smith G, Makwana N, Roberts S, Harrison PE, Laing P, Richardson JB. Early results of autologous chondrocyte implantation in the talus. J Bone Joint Surg Br. 2005;87(2):179-83.
-
28Kwak SK, Kern BS, Ferkel RD, Chan KW, Kasraeian S, Applegate GR. Autologous chondrocyte implantation of the ankle: 2- to 10-year results. Am J Sports Med. 2014;42:2156-64.
-
29Giannini S, Buda R, Ruffilli A, Cavallo M, Pagliazzi G, Bulzamini MC, et al. Arthroscopic autologous chondrocyte implantation in the ankle joint. Knee Surg Sports Traumatol Arthrosc. 2014;22:1311-9.
-
30Guillén-García P, Rodríguez-Iñigo E, Aráuz S, Guillén-Vicente M, Guillén-Vicente I, Caballero-Santos R, et al. Nuestra experiencia con la técnica de implante de condrocitos autólogos para el tratamiento de lesiones condrales: resultados de 50 pacientes a 2 años de seguimiento. Rev Esp Artrosc Cir Articul. 2015;22(3):120-5.
-
31Guillén-García P, Rodríguez-Íñigo E, Guillén-Vicente I, Caballero-Santos R, Guillén-Vicente M, Abelow S, et al. Increasing the Dose of Autologous Chondrocytes Improves Articular Cartilage Repair: Histological and Molecular Study in the Sheep Animal Model. Cartilage. 2014;5(2):114-22.
-
32López-Alcorocho JM, Aboli L, Guillén-Vicente I, Rodríguez-Íñigo E, Guillén-Vicente M, Fernández-Jaén TF, et al. Cartilage defect treatment using high-density autologous chondrocyte implantation: two-year follow-up. Cartilage. 2018;9(4):363-9.
-
33Grevnerts HT, Terwee CB, Kvist J. The measurement properties of the IKDC-subjective knee form. Knee Surg Sports Traumatol Arthrosc. 2015;23:3698-706.
-
34Greco NJ, Anderson AF, Mann BJ, Cole BJ, Farr J, Nissen CW, Irrgang JJ. Responsiveness of the International Knee Documentation Committee Subjective Knee Form in comparison to the Western Ontario and McMaster Universities Osteoarthritis Index, modified Cincinnati Knee Rating System, and Short Form 36 in patients with focal articular cartilage defects. Am J Sports Med. 2010;38(5):891-902.
-
35López-Alcorocho JM, Guillén-Vicente I, Rodríguez-Iñigo E, Navarro R, Caballero-Santos R, Guillén-Vicente M, et al. High-Density Autologous Chondrocyte Implantation as Treatment for Ankle Osteochondral Defects. Cartilage. 2019 Mar 17:1947603519835898.
-
36Cook JJ, Cook EA, Rosenblum BI, Landsman AS, Roukis TS. Validation of the American College of Foot and Ankle Surgeons Scoring Scales. J Foot Ankle Surg. 2011;50:420-9.
Descargar artículo:

Licencia:
Este contenido es de acceso abierto (Open-Access) y se ha distribuido bajo los términos de la licencia Creative Commons CC BY-NC-ND (Reconocimiento-NoComercial-SinObraDerivada 4.0 Internacional) que permite usar, distribuir y reproducir en cualquier medio siempre que se citen a los autores y no se utilice para fines comerciales ni para hacer obras derivadas.
Comparte este contenido
En esta edición
- Update on the treatment of focal cartilage lesions of the knee
- Comparison of the intraarticular injection of platelet rich plasma (PRGF®) and hyaluronic acid (Hyalone®) in the treatment of chondral lesions: a randomized, prospective clinical study
- Microfractures or bone marrow stimulation (BMS): evolution of the technique
- Fresh osteochondral graft. Indications, surgical technique and scientific evidence
- Autologous matrix-induced chondrogenesis (AMIC)
- Instant CEMTRO Cell (ICC), high density autologous chondrocytes implantation
- Regeneration of joint cartilage: perspectives and future
- Autologous chondrocyte implant surgery of the knee
- Full thickness chondral ulcers of the patella and femoral condyle of the knee
Más en PUBMED
Más en Google Scholar
Más en ORCID
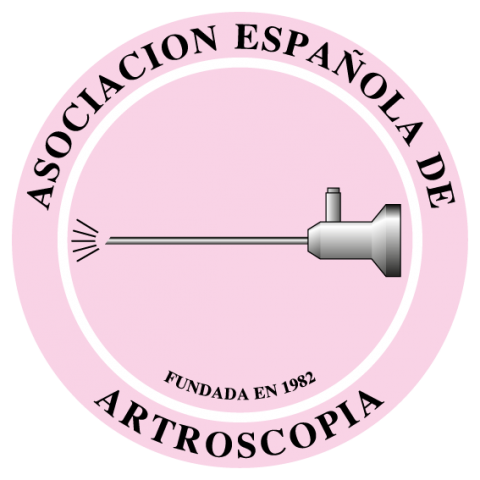

Revista Española de Artroscopia y Cirugía Articular está distribuida bajo una licencia de Creative Commons Reconocimiento-NoComercial-SinObraDerivada 4.0 Internacional.