Autologous matrix-induced chondrogenesis (AMIC)
Condrogénesis inducida por matrices (AMIC)
Resumen:
Objetivo: examinar la evolución y la situación actual de la técnica AMIC y conocer los principios biológicos sobre los que se sustenta, así como los resultados de su utilización en el tratamiento de las lesiones condrales.
Método: se realiza una revisión de la bibliografía sobre la ciencia básica relativa a las lesiones de cartílago, la técnica quirúrgica y los resultados clínicos respecto a otras técnicas de reparación y regeneración condral.
Resultados: en los estudios publicados, la técnica AMIC consigue mejoría del dolor y de las escalas clínicas y funcionales, obteniendo mejores resultados que las microfracturas y similares a otras técnicas reparativas.
Conclusiones: la condrogénesis inducida por matrices es una técnica segura, técnicamente reproducible y efectiva para el tratamiento de las lesiones de cartílago, con buenos resultados clínicos para los pacientes. No obstante, hacen falta estudios a largo plazo para conocer su evolución con el tiempo.
Nivel de evidencia: 5.
Relevancia clínica: es importante conocer una de las opciones de que disponemos para el tratamiento de las lesiones condrales. Dentro del abanico de tratamientos que existen, la técnica AMIC es relativamente novedosa y con resultados prometedores.
Abstract:
Objective: To analyze the evolution and current status of the autologous matrix-induced chondrogenesis (AMIC) technique and know its underlying biological principles, as well as the results of its use in treating chondral lesions.
Method: A literature review is made of the basic science referred to cartilage injuries, the surgical technique, and clinical outcomes versus other chondral repair and regeneration techniques.
Results: According to the published studies, AMIC improves pain and the clinical and functional scores, affording better outcomes than microfracture surgery and with results similar to those of other repair techniques.
Conclusions: Matrix-induced chondrogenesis is a safe, technically reproducible and effective technique for the treatment of cartilage injuries, with good clinical outcomes for the patients. However, long-term studies are needed to assess the evolution of the results over time.
Level of evidence: 5.
Clinical relevance: It is important to know one of the options available for the treatment of chondral lesions. Within the range of existing treatments, AMIC is a relatively novel technique affording promising results.
Introduction
The treatment of joint cartilage defects constitutes one of the main challenges in current orthopedic surgery. The reported incidence of chondral lesions among individuals between 40-50 years of age is 60%(1). These defects give rise to alterations that may result in considerable pain and functional limitation, and moreover pose a risk of osteoarthrosis over the long term(2,3). In addition, it has been seen that such patients present clinical situations similar to those of individuals with osteoarthrosis in wait of total knee replacement surgery, with even poorer clinical scores than those in wait of anterior cruciate ligament reconstruction(4). All this is attributable to the high specialization of cartilage tissue, allowing correct load transmission and affording a uniform joint contact surface. Nevertheless, repair potential is very limited, due to the avascular nature and low mitotic activity of the chondrocytes(5).
The aim of most treatments is to create a congruent joint surface with competent biomechanical properties, restore joint function, and prevent degeneration to osteoarthrosis. Such surgical treatment methods include repair techniques, regenerative procedures and palliative techniques(6). Repair techniques involve the use of tissue histologically different from cartilage, while regenerative procedures are characterized by complete replacement of the damaged tissue, maintaining the same architecture and biomechanical properties.
The repair techniques include bone marrow stimulation strategies, which have been in use since 1959, when Pridie(7) introduced subchondral perforation surgery. This technique was subsequently improved upon by Steadman(8) with microfracture surgery, in which motor-driven perforation was eliminated in order to avoid heat-induced necrosis. This technique exposes the chondral lesions to mesenchymal stromal cells ( MSCs) from the bone marrow. This exposure to cells and growth factors forms a clot that induces lesion repair(9). An important element introduced by Steadman is the correct preparation of the damaged zone through curettage of the calcified cartilage layer and removal of all the pathological cartilage tissue to the transition to healthy cartilage, in order to secure a bed better able to accommodate the mentioned clot.
At present, the nanofracture technique has been developed thanks to improved understanding of the architecture and function of subchondral bone. The mentioned technique uses a smaller diameter needle allowing better penetration of the subchondral bone, without compacting the margins of the perforations. This favours greater preservation of the trabecular structure, with posterior remodelling more similar to native subchondral bone(10,11). In addition, in vivo studies have shown that the histological qualities of the cartilage obtained are more similar to hyaline cartilage than those resulting from microfracture surgery(12,13).
Behrens(14,15) introduced autologous matrix-induced chondrogenesis (AMIC) - a technique that combines microfractures with the application of a biodegradable type I/III collagen membrane that stabilizes and protects the clot formed by the microfractures. The matrix or scaffold is affixed to the cartilage defect by means of sutures or fibrin glue. This procedure can be carried out via a miniarthrotomy or adopting a fully arthroscopic approach, and moreover offers the advantage of requiring a single surgical step.
In recent years there has been an increase in the number of AMIC-like procedures, with variations in the surgical technique and type of matrix employed. There are promising lines of research on the addition of growth factors to the matrix, resulting in selective recruitment and stimulation of the mesenchymal cells(16,17,18). Such factors moreover could activate the chondrocytes surrounding the healthy cartilage to fill the osteochondral defect. The purpose of the present study was to review the underlying biological principles, the surgical technique, and the current clinical status of matrix-induced chondrogenesis.
Basic science
Mesenchymal stem cells, MSC, are progenitor cells found in a great variety of adult tissues, including the bone marrow(19). These cells are able to differentiate towards the formation of mesodermal tissues, including cartilage, bone and adipose tissue(20). In order for this to occur, however, correct interaction among the MSCs, the extracellular matrix (ECM) proteins and growth factors (GFs) is essential(21). Tallheden(22) observed that MSCs from microfractures exhibited the same phenotypic plasticity as the chondrogenic cells of the basal substrate. Kramer in turn demonstrated that collagen matrixes satisfactorily house the cells from the bone marrow, since he was able to isolate MSCs from the matrixes(23).
An ideal matrix is able to imitate the biology, architecture and mechanical properties of native cartilage, with sufficient structural strength to support the mechanical requirements of the joint. It must prove stable in the tissue environment and allow cell migration and adhesion, as well as proliferation and differentiation. Furthermore, the material must be biodegradable and undergo remodelling while the new cartilage is formed and replaces the original structure, without releasing harmful degradation products and without stimulating an inflammatory response that could prove negative for tissue regeneration(24,25).
Although the AMIC technique was originally described with the use of a type I/III collagen membrane(15) (Chondro-Gide®, Geistlich Biomaterials, Wolhausen, Switzerland), different matrixes comprising different materials have been used for this procedure. These implants may be made of natural or synthetic polymers, as well as of mixed compounds (Table 1). The most widely used natural polymers include collagen, hyaluronic acid, chitosan (Figure 1), agarose and alginate, and are characterized by great biocompatibility and biodegradability. Likewise, their surfaces contain ligands that are recognized by cell receptors, thereby facilitating adaptation(26). On the other hand, synthetic polymers such as polylactic acid or polyglycolic acid are characterized by mechanical resistance, a lack of immunogenicity, and a zero risk of pathogen transmission(25,26).
reaca.28171.fs1907031en-figure1.png
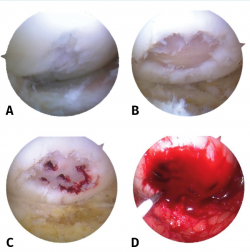
Figure 1. View of the right knee from the anteromedial port. Chondral lesion measuring 10 × 10 mm in the external femoral condyle. A: Outerbridge grade II/III chondral lesion; B: lesion curettage with harvesting of subchondral bone; C: performance of microfractures; D: placement of the chitosan matrix (scaffold).
Type I/III collagen-based matrixes are the most widely used option, and are cited in a greater number of publications(27,28,29,30). These matrixes are structured as a double layer, with a porous surface allowing MSC fixation to the collagen fibers for cell proliferation and differentiation, and a non-porous smooth surface that retains the clot within the defect. This type of matrix is reabsorbed within 6-24 weeks after implantation. Gille(31) demonstrated in his study that the cells that develop in the membrane form a multilayer structure with cells similar to chondrocytes. Gigante(32) obtained biopsies from 5 patients previously subjected to treatment for chondral lesions and recorded a mean histological score (International Cartilage Repair Society [ICRS]) of 60 - where a score of 100 indicates maximum similarity to joint cartilage - and also described an arthroscopic visual appearance similar to that of healthy cartilage.
Absorbable polyglycolic acid (PGA) matrixes treated with hyaluronic acid are also available, acting as a sponge, retaining the clot and MSCs within the chondral defect(33). Animal models have shown that hyaline cartilage of greater quality is formed with the use of this type of implant than with microfracture surgery alone(34,35); likewise, hyaluronic acid has been seen to induce chondrogenic differentiation of the MSCs(36,37). The mechanical stability of this matrix allows for easy handling during surgery and safe fixation with both sutures and fibrin glue, and reabsorbable pins. To date, its presence in the literature is limited to case reports(33,38) and case series involving few patients(39,40).
Implants composed of semisynthetic derivatives of hyaluronic acid can also be found, structured into randomly distributed fibers, with no particular predominant direction. This facilitates cell contact and extracellular matrix formation, as well as MSC retention. Buda(41) described their use in 2010, combining the AMIC technique with bone marrow MSCs obtained from the centrifugation of iliac crest marrow aspirate and platelet rich plasma (PRP). These membranes are impregnated with the MSC concentrate and are placed in the defect, followed by the application of PRP supplying growth factors. Vannini(42) reproduced the technique in 6 patients with osteochondritis dissecans, with good results.
Surgical technique
The original surgical technique was described by Benthiem and Behrens(14,15) in application to chondral defects of the knee. The indications of the technique are chondral or osteochondral lesions measuring under 1.5 cm2 in size. In the case of osteochondral lesions, the authors filled the bone defect with cancellous bone from the proximal tibia, compacting it and placing the membrane upon the grafted bone. The contraindications of the technique are mirror lesions or lesions of an inflammatory origin; damage to the collateral ligaments or cruciate ligaments; deficits in extension of over 10º or in flexion of over 100º; poor alignment of the lower extremity; and associated fractures.
The procedure can be performed under spinal or general anesthesia. The patient is placed in supine decubitus, and the use of tourniquet ischemia is advised. In a first step, arthroscopy of the affected joint is carried out to check the size and location of the defect, and to diagnose possible associated lesions. If the criteria for AMIC are met, the authors recommend a minimally invasive arthrotomy. Under direct visualization of the lesion, curettage of the defective cartilage tissue is performed until healthy cartilage is reached, with removal of the layer of calcified cartilage until subchondral tissue appears. The microfractures technique is then carried out as described by Steadman(8).
Once this process is completed, the final size of the chondral defect is determined to calculate the dimensions of the membrane to be placed. It is advisable to use a template to transfer the correct size to the matrix. Some surgeons use aluminium or plastic sheets for this purpose. The authors suggest the choice of a size slightly smaller than that of the defect, in order to avoid its displacement with joint motion.
The next step involves membrane fixation. The fixation method preferred by Behrens comprises the use of partially autologous fibrin glue obtained from the centrifugation of peripheral blood of the patient, and mixing it with exogenous fibrinogen. It is also possible to use commercial fibrin formulations. It is advisable to position the membrane slightly below the level of the cartilage in order to avoid posterior displacement (Figure 2).
reaca.28171.fs1907031en-figure2.png
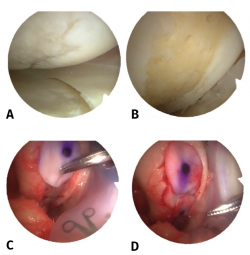
Figure 2. View of the right knee from the anteroexternal port. Chondral lesion in the internal femoral condyle measuring 10 × 20 mm. A: Outerbridge grade III chondral lesion; B: lesion cleared to the subchondral bone and performance of microfractures; C: placement of the type I/III collagen matrix; D: final view with complete covering of the chondral defect.
With regard to postoperative management, some authors recommend knee immobilization during 7 days in extension(43). Others keep the operated extremity without weight bearing for a period of 2-6 weeks(41,42,43,44). Most surgeons allow impact-generating activities after 6 months and sports activities from one year after surgery. The authors describing the original technique allowed maximum partial weight bearing of 15-20 kg during 6 weeks. Furthermore, maximum flexion of the knee is limited to 30º during two weeks, with progressive increments to 60º and 90º from week 4 and 6, respectively, with the incorporation of joint range and muscle strengthening exercises.
Purely arthroscopic techniques have been described in recent years. In 2012, Piontek(45) described a variation of the technique in which curettage is followed by the use of trephines to determine the size of the lesion. Subsequently, under dry arthroscopy conditions, subchondral perforations are made, followed by the placement of a Chondro-Gide® membrane in the defect. Lastly, the membrane is covered with commercial fibrin glue (Tissucol®, Baxter, Warsaw, Poland), and stability is checked by performing flexion-extension movements. The joint range over which the lesion remains without bearing weight is then recorded to determine the safe mobility range for rehabilitation. In order to avoid displacement of the membrane, drains are not used.
In 2015, Sadlik(46) described an arthroscopic technique for the treatment of patellar lesions. A retracting plate is used, bound to extra-articular sutures, and placed under the medial patellar retinaculum - allowing elevation and inclination of the patella in order to better visualize the lesion and maintain the operating space in the dry step of arthroscopy. In addition, a guide and introducer are used to insert the matrix and position it correctly over the chondral defect.
Schagemann(47), in a study of 50 patients, compared the arthroscopic technique versus the miniarthrotomy procedure, and concluded that there are no significant differences between them in terms of the outcomes. However, the decision to choose one technique or the other should be based on surgeon skill.
Different arthroscopic techniques have been proposed to treat chondral lesions in other joints such as the ankle(48,49), hip(50) and shoulder(51).
Clinical evidence
Buda(41) performed the AMIC technique in 20 patients using Hyalofast® together with MSCs and PRP, and recorded statistically significant improvements in the International Knee Documentation Committee (IKDC) score and Knee Injury and Osteoarthritis Outcome Score (KOOS). At magnetic resonance imaging (MRI) controls after 12 and 24 months, improvements were recorded in the Magnetic Resonance Observation of Cartilage Repair Tissue (MOCART) score, and a statistically significant relationship was observed between the KOOS at 24 months and MRI signal intensity. The histological study revealed a matrix rich in proteoglycans, an almost regular surface layer with the presence of cells uniformly distributed within the tissue, and type II collagen positivity throughout the thickness of the biopsy sample.
Vannini(42) used the same technique in 6 patients between 14-18 years of age with osteochondritis dissecans and a mean defect size of 4.6 ± 1.5 cm2. The same satisfactory clinical outcomes were obtained, with an increase in IKDC score, but no significant correlation was observed between the MOCART parameters and the clinical scales.
In 2012, Kusano(27) published the results corresponding to 38 treated patients with single lesions over 2 cm2 in size and aged under 50 years, using Chondro-Gide®. Significant increases were recorded in the IKDC and Lysholm scales, but the MOCART score was not correlated to the clinical outcomes, since most of the lesions showed incomplete defect repair, with damaged repair tissue and a heterogeneous structure, as well as an altered subchondral plate.
Pascarella(52) modified the technique seeking to increase the MSC load. Perforations were made instead of microfractures, and the Chondro-Gide® matrix was impregnated with bone marrow concentrate obtained directly from aspiration through one of the perforations. The clinical outcomes (IKDC and Lysholm scales) revealed increases similar to those obtained by Kusano(27), and the postoperative MRI study showed a significant decrease in the area of the defect and in subchondral edema in 53% of the patients.
In 2010, Gille(43) published the results of 32 chondral lesions treated with type I/III collagen membranes in 27 patients between 16-50 years of age, with a mean defect size of 4.2 cm2 and subjected to a minimum follow-up of two years. A significant increase in the Lysholm score and in the ICRS scale was observed during the first 24 months of follow-up, with a decrease from two years onwards. In 2012(28), as part of the AMIC Registry, the sample was expanded to 57 patients, with the observation of a significant increase in the Lysholm score and in the pain visual analogue scale (VAS).
A more recent study involving 21 patients with a mean follow-up period of 7 years recorded a significant increase in the IKDC and Lysholm scores throughout follow-up(53). Furthermore, no association was observed between patient age and body mass index (BMI) and the functional indices. A decrease in the area of the defect and in subchondral edema was also recorded in 66% of the patients.
With regard to ankle joint lesions, significant improvements have been observed in the VAS score and in the ankle score of the American Orthopedic Foot and Ankle Society (AOFAS), in the studies published by D'ambrosi(54,55), Usuelli(48) and Valderrábano(56,57). Likewise, MRI assessment of the cartilage revealed an increase in the MOCART score(48,56).
A number of studies have reported improvements in the modified Harris Hip Score (mHHS) in chondral defects of the hip(58,59,60). Fontana(58) compared the AMIC technique with microfracture surgery in acetabular lesions caused by femoroacetabular impingement (FAI), and found the clinical improvement to remain stable over the 5 years of follow-up, while in the microfractures group it was seen to gradually worsen.
Discussion
Matrix-induced chondrogenesis is a treatment option for cartilage injuries, involving the placement of a membrane capable of containing and stimulating MSCs to produce cartilage tissue. The systematic reviews carried out by Shaikh(61) and Gao(62) highlight the few existing high-quality randomized studies, due mainly to the limited number of patients and shortcomings referred to the duration of follow-up and study design. This is particularly manifest in the case of those studies that establish comparisons with other repair techniques. No clinical trials to date have compared AMIC versus autologous chondrocyte implantation (ACI) in the knee or ankle, and likewise no comparative trials have been made with microfracture surgery in the latter.
In contrast, randomized studies have compared AMIC versus microfracture surgery alone(63,64). Anders(64) compared 38 patients with lesions presenting a mean size of 3.4 cm2 and 37 years old on average, with a follow-up of 2 years. A significant increase was observed in ICRS scale and in the modified Cincinnati scale for both microfractures and for the AMIC group at one year of follow-up, with maintenance of the increase after two years. There were no significant differences between the groups. Volz(63) recorded similar results after two years. However, after 5 years there was a significant decrease in the clinical outcomes in the microfractures group, while the patients in the AMIC group maintained or even improved their outcomes.
The ACI technique involves the harvesting of cartilage samples from a non-weight bearing or chondral lesion donor zone, followed by processing in the laboratory to release and culture the chondrocytes. In second step surgery, these chondrocytes are implanted in the defect. The third generation of this process - matrix-induced autologous chondrocyte implantation (MACI) - includes the use of a membrane to retain the chondrocytes within the lesion. To our knowledge, only one study has compared AMIC versus MACI to date(60). In the mentioned study, Mancini(60), in patients with hip lesions measuring 2-4 cm2 in size, compared AMIC versus MACI and concluded that both groups showed clinical improvement, though without significant differences over the 5 years of follow-up. However, the study lacked a randomized design and did not contribute MRI data through MOCART.
The literature does offer high-quality studies establishing comparisons with other techniques such as microfracture surgery(65,66) and mosaicplasty(67). From the results obtained, it can be deduced that the AMIC technique yields results similar to those of ACI, with the advantage that AMIC requires only single surgery, and the structure and logistics of a laboratory are not needed.
Because of the relative novelty of this treatment, few studies reporting long-term outcomes are available. The identified studies with the longest follow-up involved a period of 5 years(43,56,58,60). Gille(43) reported deterioration of the clinical outcomes after 18-24 months as assessed by the ICRS, Lysholm and Tegner scales. Anders in turn reported a tendency towards stabilization of the clinical outcomes from 12 months, and 11% of the patients even experienced a worsening of the clinical parameters. However, Fontana(58) and Mancini(60) recorded good results that persisted over the 5 years of the studies - though all the patients had undergone additional operations for the treatment of femoroacetabular impingement (FAI).
A number of studies have included additional surgeries associated to treatment of the chondral lesions, such as realignment osteotomies(27,41,43,56,68) or the treatment of femoroacetabular impingement (FAI)(58,60) - making it difficult to determine whether the clinical effect is a result of repair of the cartilage defect, the associated surgeries, or both. Only Kusano(27) compared patients treated exclusively with the AMIC technique versus patients in which realignment osteotomy was added. No significant differences in outcome were observed. Because of this variability in terms of the associated treatments, it is difficult to compare the rehabilitation protocols and determine which is best.
To date, no studies have reported serious adverse effects related to this technique. With regard to repeat surgeries, these have been limited to the elimination of intralesional osteophytes(29,68), often in relation to the microfracture procedure.
In this regard, several microfracture techniques have been used as a first step for AMIC. These range from the use of punches(27,43,58,60,64) to driving motors(56,58), though no comparative studies have established which approach is best for performing microfractures in the context of AMIC. Nevertheless, increased quality cartilage has been recorded with the use of nanofractures versus microfractures in studies conducted in vivo(12,13).
Another key technical aspect is matrix fixation. This can be done using sutures, fibrin glue or subchondral anchoring. Sutures and fibrin afford sufficient membrane stability(69), although the use of sutures is not only technically more demanding but also causes local damage to healthy cartilage similar to that seen in the early stages of osteoarthrosis(70). More recent studies show subchondral anchoring to offer the greatest mechanical resistance(71). The studies of Volz(63)and Anders(64) compared two groups of patients subjected to suture fixation versus another group in which fixation was made with fibrin glue - no significant clinical or radiological differences being found between them.
With regard to matrix composition, Welsch(72) found the repair tissue to be better with collagen matrixes than with matrixes based on hyaluronic acid, according to radiological criteria. This is in contrast to studies suggesting that hyaluronic acid is able to induce chondrogenesis(73,74). Wu(74) showed the addition of hyaluronic acid to polylactic-co-glycolic acid (PLGA) matrixes to yield greater levels of type II collagen and glycosaminoglycans. Mechanically, polyglycolic acid (PGA) matrixes have been shown to support greater loads, independently of the fixation used, compared with collagen membranes and PLGA membranes(31).
On the other hand, in a recent systematic review, Gao(75) concluded that there is not enough evidence that biomaterials composed of type I/III collagen can by themselves induce chondrogenesis. Indeed, in vitro studies indicated that type II collagen matrixes induce greater cell proliferation and a greater accumulation of collagen and glycosaminoglycans than type I collagen matrixes(76).
Conclusions
Matrix-induced chondrogenesis is a safe, technically reproducible and effective technique for the treatment of cartilage injuries, with good clinical outcomes for the patients. Furthermore, it has the advantage of being applicable in a single surgical step and without the morbidity associated with the creation of a donor zone - in contrast to ACI or mosaicplasty. Nevertheless, randomized studies involving larger patient samples and improved methodological designs are needed to establish comparisons with the rest of the existing therapeutic options. Likewise, long-term studies are required to determine whether the technique is able to avoid or delay joint degeneration.
Tablas
Figuras
Figure 1. View of the right knee from the anteromedial port. Chondral lesion measuring 10 × 10 mm in the external femoral condyle. A: Outerbridge grade II/III chondral lesion; B: lesion curettage with harvesting of subchondral bone; C: performance of microfractures; D: placement of the chitosan matrix (scaffold).
Figure 2. View of the right knee from the anteroexternal port. Chondral lesion in the internal femoral condyle measuring 10 × 20 mm. A: Outerbridge grade III chondral lesion; B: lesion cleared to the subchondral bone and performance of microfractures; C: placement of the type I/III collagen matrix; D: final view with complete covering of the chondral defect.
Información del artículo
Cita bibliográfica
Autores
Eduardo Sánchez Alepuz
Servicio de Ortopedia y Traumatología. Hospital IMED. Valencia
Joan Part Soriano
Servicio de Cirugía Ortopédica y Traumatología. Unión de Mutuas. Valencia
Grupo de Estudio e Investigación del Cartílago Articular de Valencia (GEICAV)
Ignacio Peregrín Nevado
Servicio de Cirugía Ortopédica y Traumatología. Hospital IMED Valencia. Burjassot, Valencia
Grupo de Estudio e Investigación del Cartílago Articular de Valencia (GEICAV)
Javier Zurriaga Carda
Servicio de Cirugía Ortopédica y Traumatología. Hospital IMED Valencia. Burjassot, Valencia
Grupo de Estudio e Investigación del Cartílago Articular de Valencia (GEICAV)
Jean Maria Gómez Alessandri
Servicio de Cirugía Ortopédica y Traumatología. Unión de Mutuas. Valencia
Grupo de Estudio e Investigación del Cartílago Articular de Valencia (GEICAV)
Jose Vicente Díaz
Servicio de Cirugía Ortopédica y Traumatología. IMED Valencia. Burjassot, Valencia
Grupo de Estudio e Investigación del Cartílago Articular de Valencia (GEICAV)
Rafael Calero Ferrandiz
Servicio de Cirugía Ortopédica y Traumatología. Unión de Mutuas. Valencia
Grupo de Estudio e Investigación del Cartílago Articular de Valencia (GEICAV)
Ethical responsibilities
Conflicts of interest. The authors declare that they have no conflicts of interest.
Financial support. This study has received no financial support.
Protection of people and animals. The authors declare that this research has not involved studies in humans or in animals.
Data confidentiality. The authors declare that the protocols of their centre referred to the publication of patient information have been followed.
Right to privacy and informed consent. The authors declare that no patient data appear in this article.
Referencias bibliográficas
-
1Widuchowski W, Widuchowski J, Trzaska T. Articular cartilage defects: Study of 25,124 knee arthroscopies. Knee. 2007;14(3):177-82.
-
2Alford JW, Cole BJ. Cartilage Restoration, Part 1: Basic Science, Historical Perspective, Patient Evaluation, and Treatment Options. Am J Sports Med. 2005;33(2):295-306.
-
3Mankin HJ. The response of articular cartilage to mechanical injury. J Bone Joint Surg Am. 1982;64(3):460-6.
-
4Heir S, Nerhus TK, Røtterud JH, Løken S, Ekeland A, Engebretsen L, Arøen A. Focal Cartilage Defects in the Knee Impair Quality of Life as Much as Severe Osteoarthritis: A Comparison of Knee Injury and Osteoarthritis Outcome Score in 4 Patient Categories Scheduled for Knee Surgery. Am J Sports Med. 2009;38(2):231-7.
-
5Gomoll AH, Minas T. The quality of healing: articular cartilage. Wound Repair Regen. 2014;22:30-8.
-
6Zylińska B, Silmanowicz P, Sobczyńska-Rak A, Jarosz Ł, Szponder T. Treatment of articular cartilage defects: focus on tissue engineering. In Vivo. 2018;32(6):1289-300.
-
7Pridie KH. A method of resurfacing osteoarthritic knee joints. J Bone Joint Surg. 1959:618-9.
-
8Steadman JR, Rodkey WG, Rodrigo JJ. Microfracture: surgical technique and rehabilitation to treat chondral defects. Clin Orthop Relat Res. 2001;(391 Suppl):S362-9.
-
9Steadman JR, Rodkey WG, Briggs KK. Microfracture to treat full-thickness chondral defects: surgical technique, rehabilitation, and outcomes. J Knee Surg. 2002;15(3):170-6.
-
10Zedde P, Cudoni S, Manunta L, Passino ES, Masala G, Brunetti A, et al. Second generation needling techniques for the treatment of chondral defects in animal model. Joints. 2017;5(1):27-33.
-
11Zedde P, Cudoni S, Giachetti G, Manunta ML, Masala G, Brunetti A, Manunta AF. Subchondral bone remodeling: Comparing nanofracture with microfracture. An ovine in vivo study. Joints. 2016;4(2):87-93.
-
12Chen H, Hoemann CD, Sun J, Chevrier A, McKee MD, Shive MS, et al. Depth of subchondral perforation influences the outcome of bone marrow stimulation cartilage repair. J Orthop Res. 2011;29(8):1178-4.
-
13Orth P, Duffner J, Zurakowski D, Cucchiarini M, Madry H. Small-Diameter Awls Improve Articular Cartilage Repair After Microfracture Treatment in a Translational Animal Model. Am J Sports Med. 2016;44(1):209-19.
-
14Benthien JP, Behrens P. The treatment of chondral and osteochondral defects of the knee with autologous matrix-induced chondrogenesis (AMIC): method description and recent developments. Knee Surg Sport Traumatol Arthrosc. 2011;19(8):1316-9.
-
15Benthien JP, Behrens P. Autologous matrix-induced chondrogenesis (AMIC): combining microfracturing and a collagen I/III matrix for articular cartilage resurfacing. Cartilage. 2010;1(1):65-8.
-
16Ge Z, Li C, Heng BC, Cao G, Yang Z. Functional biomaterials for cartilage regeneration. J Biomed Mater Res A. 2012 Sep;100(9):2526-36.
-
17Hsieh YH, Hsieh MF, Fang CH, Jiang CP, Lin B, Lee HM. Osteochondral Regeneration Induced by TGF-β Loaded Photo Cross-Linked Hyaluronic Acid Hydrogel Infiltrated in Fused Deposition-Manufactured Composite Scaffold of Hydroxyapatite and Poly (Ethylene Glycol)-Block-Poly(ε-Caprolactone). Polymers (Basel). 2017;9(12):182.
-
18Bian L, Zhai DY, Tous E, Rai R, Mauck RL, Burdick JA. Enhanced MSC chondrogenesis following delivery of TGF-beta3 from alginate microspheres within hyaluronic acid hydrogels in vitro and in vivo. Biomaterials. 2011;32(27):6425-34.
-
19Yoo JU, Barthel TS, Nishimura K, Solchaga L, Caplan AI, Goldberg VM, Johnstone B. The chondrogenic potential of human bone-marrow-derived mesenchymal progenitor cells. J Bone Joint Surg Am. 1998 Dec;80(12):1745-57.
-
20Mackay AM, Beck SC, Murphy JM, Barry FP, Chichester CO, Pittenger MF. Chondrogenic differentiation of cultured human mesenchymal stem cells from marrow. Tissue Eng. 1998;4(4):415-28.
-
21Van der Kraan PM, Buma P, van Kuppevelt T, van den Berg WB. Interaction of chondrocytes, extracellular matrix and growth factors: relevance for articular cartilage tissue engineering. Osteoarthr Cartil. 2002;10(8):631-7.
-
22Tallheden T, Dennis JE, Lennon DP, Sjogren-Jansson E, Caplan AI, Lindahl A. Phenotypic plasticity of human articular chondrocytes. J Bone Joint Surg Am. 2003;85-A Suppl:93-100.
-
23Kramer J, Bohrnsen F, Lindner U, Behrens P, Schlenke P, Rohwedel J. In vivo matrix-guided human mesenchymal stem cells. Cell Mol Life Sci. 2006;63(5):616-26.
-
24Żylińska B, Silmanowicz P, Sobczyńska-Rak A, Jarosz Ł, Szponder T. Treatment of Articular Cartilage Defects: focus on Tissue Engineering. In Vivo. 2018 Nov-Dec;32(6):1289-300.
-
25Fahmy MD, Shah B, Razavi M, et al. Smart Biomaterials for Tissue Engineering of Cartilage. RSC Smart Mater. 2017;2017(25):194-229.
-
26Chiang H, Jiang CC. Repair of articular cartilage defects: review and perspectives. J Formos Med Assoc. 2009;108(2):87-101.
-
27Kusano T, Jakob RP, Gautier E, Magnussen RA, Hoogewoud H, Jacobi M. Treatment of isolated chondral and osteochondral defects in the knee by autologous matrix-induced chondrogenesis (AMIC). Knee Surg Sport Traumatol Arthrosc. 2012;20(10):2109-15.
-
28Gille J, Behrens P, Volpi P, de Girolamo L, Reiss E, Zoch W, Anders S. Outcome of Autologous Matrix Induced Chondrogenesis (AMIC) in cartilage knee surgery: data of the AMIC Registry. Arch Orthop Trauma Surg. 2013 Jan;133(1):87-93.
-
29Dhollander A, Moens K, Van der Maas J, Verdonk P, Almqvist KF, Victor J. Treatment of patellofemoral cartilage defects in the knee by autologous matrix-induced chondrogenesis (AMIC). Acta Orthop Belg. 2014;80(2):251-9.
-
30Sadlik B, Puszkarz M, Kosmalska L, Wiewiorski M. All-Arthroscopic Autologous Matrix-Induced Chondrogenesis-Aided Repair of a Patellar Cartilage Defect Using Dry Arthroscopy and a Retraction System. J Knee Surg. 2017;30(9):925-9.
-
31Gille J, Meisner U, Ehlers EM, Muller A, Russlies M, Behrens P. Migration pattern, morphology and viability of cells suspended in or sealed with fibrin glue: a histomorphologic study. Tissue Cell. 2005;37(5):339-48.
-
32Gigante A, Calcagno S, Cecconi S, Ramazzotti D, Manzotti S, Enea D. Use of collagen scaffold and autologous bone marrow concentrate as a one-step cartilage repair in the knee: histological results of second-look biopsies at 1 year follow-up. Int J Immunopathol Pharmacol. 2011;24(1 Suppl 2):69-72.
-
33Patrascu JM, Freymann U, Kaps C, Poenaru DV. Repair of a post-traumatic cartilage defect with a cell-free polymer-based cartilage implant. J Bone Joint Surg Br. 2010;92-B(8):1160-3.
-
34Erggelet C, Endres M, Neumann K, Morawietz L, Ringe J, Haberstroh K, et al. Formation of cartilage repair tissue in articular cartilage defects pretreated with microfracture and covered with cell-free polymer-based implants. J Orthop Res. 2009;27(10):1353-60.
-
35Erggelet C, Neumann K, Endres M, Haberstroh K, Sittinger M, Kaps C. Regeneration of ovine articular cartilage defects by cell-free polymer-based implants. Biomaterials. 2007;28(36):5570-80.
-
36Hegewald AA, Ringe J, Bartel J, Krüger I, Notter M, Barnewitz D, et al. Hyaluronic acid and autologous synovial fluid induce chondrogenic differentiation of equine mesenchymal stem cells: a preliminary study. Tissue Cell. 2004;36(6):431-8.
-
37Saw KY, Anz A, Merican S, Tay YG, Ragavanaidu K, Jee CS, McGuire DA. Articular cartilage regeneration with autologous peripheral blood progenitor cells and hyaluronic acid after arthroscopic subchondral drilling: a report of 5 cases with histology. Arthroscopy. 2011 Apr;27(4):493-506.
-
38Zantop T, Petersen W. Arthroscopic implantation of a matrix to cover large chondral defect during microfracture. Arthroscopy. 2009 Nov;25(11):1354-60.
-
39Becher C, Ettinger M, Ezechieli M, Kaps C, Ewig M, Smith T. Repair of retropatellar cartilage defects in the knee with microfracture and a cell-free polymer-based implant. Arch Orthop Trauma Surg. 2015;135(7):1003-10.
-
40Dhollander AAM, Verdonk PCM, Lambrecht S, Almqvist KF, Elewaut D, Verbruggen G, Verdonk R. The combination of microfracture and a cell-free polymer-based implant immersed with autologous serum for cartilage defect coverage. Knee Surg Sport Traumatol Arthrosc. 2012;20(9):1773-80.
-
41Buda R, Vannini F, Cavallo M, Grigolo B, Cenacchi A, Giannini S. Osteochondral lesions of the knee: a new one-step repair technique with bone-marrow-derived cells. J Bone Joint Surg Am. 2010 Dec;92 Suppl 2:2-11.
-
42Vannini F, Battaglia M, Buda R, Cavallo M, Giannini S. “One Step” Treatment of Juvenile Osteochondritis Dissecans in the Knee: Clinical Results and T2 Mapping Characterization. Orthop Clin North Am. 2012;43(2):237-44.
-
43Gille J, Schuseil E, Wimmer J, Gellissen J, Schulz AP, Behrens P. Mid-term results of Autologous Matrix-Induced Chondrogenesis for treatment of focal cartilage defects in the knee. Knee Surg Sport Traumatol Arthrosc. 2010;18(11):1456-64.
-
44Dhollander AA, Verdonk PC, Lambrecht S, Verdonk R, Elewaut D, Verbruggen G, Almqvist KF. Midterm results of the treatment of cartilage defects in the knee using alginate beads containing human mature allogenic chondrocytes. Am J Sports Med. 2012 Jan;40(1):75-82.
-
45Piontek T, Ciemniewska-Gorzela K, Szulc A, Naczk J, Słomczykowski M. All-arthroscopic AMIC procedure for repair of cartilage defects of the knee. Knee Surg Sport Traumatol Arthrosc. 2012;20(5):922-5.
-
46Sadlik B, Wiewiorski M. Implantation of a collagen matrix for an AMIC repair during dry arthroscopy. Knee Surg Sport Traumatol Arthrosc. 2015;23(8):2349-52.
-
47Schagemann J, Behrens P, Paech A, Riepenhof H, Kienast B, Mittelstädt H, Gille J. Mid-term outcome of arthroscopic AMIC for the treatment of articular cartilage defects in the knee joint is equivalent to mini-open procedures. Arch Orthop Trauma Surg. 2018;138(6):819-25.
-
48Usuelli FG, D’Ambrosi R, Maccario C, Boga M, de Girolamo L. All-arthroscopic AMIC® (AT-AMIC®) technique with autologous bone graft for talar osteochondral defects: clinical and radiological results. Knee Surg Sport Traumatol Arthrosc. 2018;26(3):875-81.
-
49Baumfeld T, Baumfeld D, Prado M, Nery C. All-arthroscopic AMIC® (AT-AMIC) for the treatment of talar osteochondral defects: A short follow-up case series. Foot. 2018;37(February):23-7.
-
50Fontana A. A novel technique for treating cartilage defects in the hip: a fully arthroscopic approach to using autologous matrix-induced chondrogenesis. Arthrosc Tech. 2012;1(1):e63-8.
-
51Cuéllar A, Ruiz-Ibán MA, Cuéllar R. The Use of All-Arthroscopic Autologous Matrix-Induced Chondrogenesis for the Management of Humeral and Glenoid Chondral Defects in the Shoulder. Arthrosc Tech. 2016;5(2):e223-e227.
-
52Pascarella A, Ciatti R, Pascarella F, Latte C, Di Salvatore MG, Liguori L, Iannella G. et al. Treatment of articular cartilage lesions of the knee joint using a modified AMIC technique. Knee Surg Sport Traumatol Arthrosc. 2010;18(4):509-13.
-
53Schiavone Panni A, Del Regno C, Mazzitelli G, D’Apolito R, Corona K, Vasso M. Good clinical results with autologous matrix-induced chondrogenesis (Amic) technique in large knee chondral defects. Knee Surg Sport Traumatol Arthrosc. 2018;26(4):1130-6.
-
54D’Ambrosi R, Maccario C, Ursino C, Serra N, Usuelli FG. Combining Microfractures, Autologous Bone Graft, and Autologous Matrix-Induced Chondrogenesis for the Treatment of Juvenile Osteochondral Talar Lesions. Foot Ankle Int. 2017;38(5):485-95.
-
55D’Ambrosi R, Maccario C, Serra N, Liuni F, Usuelli FG. Osteochondral Lesions of the Talus and Autologous Matrix-Induced Chondrogenesis: Is Age a Negative Predictor Outcome? Arthroscopy. 2017;33(2):428-35.
-
56Valderrábano V, Miska M, Leumann A, Wiewiorski M. Reconstruction of osteochondral lesions of the talus with autologous spongiosa grafts and autologous matrix-induced chondrogenesis. Am J Sports Med. 2013;41(3):519-27.
-
57Valderrábano V, Barg A, Alattar A, Wiewiorski M. Osteochondral lesions of the ankle joint in professional soccer players: treatment with autologous matrix-induced chondrogenesis. Foot Ankle Spec. 2014;7(6):522-8.
-
58Fontana A, De Girolamo L. Sustained five-year benefit of autologous matrix-induced chondrogenesis for femoral acetabular impingement-induced chondral lesions compared with microfracture treatment. Bone Joint J. 2015;97-B(5):628-35.
-
59Fontana A. Autologous Membrane Induced Chondrogenesis (AMIC) for the treatment of acetabular chondral defect. Muscles Ligaments Tendons J. 2016;6(3):367-71.
-
60Mancini D, Fontana A. Five-year results of arthroscopic techniques for the treatment of acetabular chondral lesions in femoroacetabular impingement. Int Orthop. 2014;38(10):2057-64.
-
61Shaikh N, Seah MKT, Khan WS. Systematic review on the use of autologous matrix-induced chondrogenesis for the repair of articular cartilage defects in patients. World J Orthop. 2017;8(7):588.
-
62Gao L, Orth P, Cucchiarini M, Madry H. Autologous Matrix-Induced Chondrogenesis: a Systematic Review of the Clinical Evidence. Am J Sports Med. 2019;47(1):222-31.
-
63Volz M, Schaumburger J, Frick H, Grifka J, Anders S. A randomized controlled trial demonstrating sustained benefit of Autologous Matrix-Induced Chondrogenesis over microfracture at five years. Int Orthop. 2017;41(4):797-804.
-
64Anders S, Volz M, Frick H, Gellissen J. A Randomized, Controlled Trial Comparing Autologous Matrix-Induced Chondrogenesis (AMIC®) to Microfracture: Analysis of 1- and 2-Year Follow-Up Data of 2 Centers. Open Orthop J. 2013;7(Suppl 4):133-43.
-
65Vanlauwe J, Saris DBF, Victor J, Almqvist KF, Bellemans J, Luyten FP. Five-year outcome of characterized chondrocyte implantation versus microfracture for symptomatic cartilage defects of the knee: early treatment matters. Am J Sports Med. 2011;39(12):2566-74.
-
66Saris D, Price A, Widuchowski W, Bertrand-Marchand M, Caron J, Drogset JO, et al.; SUMMIT study group. Matrix-Applied Characterized Autologous Cultured Chondrocytes Versus Microfracture: Two-Year Follow-up of a Prospective Randomized Trial. Am J Sports Med. 2014;42(6):1384-94.
-
67Bentley G, Biant LC, Vijayan S, Macmull S, Skinner JA, Carrington RWJ. Minimum ten-year results of a prospective randomised study of autologous chondrocyte implantation versus mosaicplasty for symptomatic articular cartilage lesions of the knee. J Bone Joint Surg Br. 2012;94(4):504-9.
-
68Dhollander AA, De Neve F, Almqvist KF, Verdonk R, Lambrecht S, Elewaut D, et al. Autologous matrix-induced chondrogenesis combined with platelet-rich plasma gel: technical description and a five pilot patients report. Knee Surg Sport Traumatol Arthrosc. 2011;19(4):536-42.
-
69Knecht S, Erggelet C, Endres M, Sittinger M, Kaps C, Stussi E. Mechanical testing of fixation techniques for scaffold-based tissue-engineered grafts. J Biomed Mater Res B Appl Biomater. 2007;83(1):50-7.
-
70Hunziker EB, Stahli A. Surgical suturing of articular cartilage induces osteoarthritis-like changes. Osteoarthr Cartil. 2008;16(9):1067-73.
-
71Friedman JM, Sennett ML, Bonadio MB, Orji KO, Neuwirth AL, Keah N, et al. Comparison of Fixation Techniques of 3D-Woven Poly(ϵ-Caprolactone) Scaffolds for Cartilage Repair in a Weightbearing Porcine Large Animal Model. Cartilage. 2018;9(4):428-37.
-
72Welsch GH, Mamisch TC, Zak L, Blanke M, Olk A, Marlovits S, Trattnig S. Evaluation of cartilage repair tissue after matrix-associated autologous chondrocyte transplantation using a hyaluronic-based or a collagen-based scaffold with morphological MOCART scoring and biochemical T2 mapping: preliminary results. Am J Sports Med. 2010 May;38(5):934-42.
-
73Erickson IE, Kestle SR, Zellars KH, Farrell MJ, Kim M, Burdick JA, Mauck RL. High mesenchymal stem cell seeding densities in hyaluronic acid hydrogels produce engineered cartilage with native tissue properties. Acta Biomater. 2012 Aug;8(8):3027-34.
-
74Wu SC, Chang JK, Wang CK, Wang GJ, Ho ML. Enhancement of chondrogenesis of human adipose derived stem cells in a hyaluronan-enriched microenvironment. Biomaterials. 2010;31(4):631-40.
-
75Gao L, Orth P, Cucchiarini M, Madry H. Effects of solid acellular type-I/III collagen biomaterials on in vitro and in vivo chondrogenesis of mesenchymal stem cells. Expert Rev Med Devices. 2017;14(9):717-32.
-
76Almeida HV, Sathy BN, Dudurych I, Buckley CT, O’Brien FJ, Kelly DJ. Anisotropic Shape-Memory Alginate Scaffolds Functionalized with Either Type I or Type II Collagen for Cartilage Tissue Engineering. Tissue Eng Part A. 2017;23(1-2):55-68.
Descargar artículo:

Licencia:
Este contenido es de acceso abierto (Open-Access) y se ha distribuido bajo los términos de la licencia Creative Commons CC BY-NC-ND (Reconocimiento-NoComercial-SinObraDerivada 4.0 Internacional) que permite usar, distribuir y reproducir en cualquier medio siempre que se citen a los autores y no se utilice para fines comerciales ni para hacer obras derivadas.
Comparte este contenido
En esta edición
- Update on the treatment of focal cartilage lesions of the knee
- Comparison of the intraarticular injection of platelet rich plasma (PRGF®) and hyaluronic acid (Hyalone®) in the treatment of chondral lesions: a randomized, prospective clinical study
- Microfractures or bone marrow stimulation (BMS): evolution of the technique
- Fresh osteochondral graft. Indications, surgical technique and scientific evidence
- Autologous matrix-induced chondrogenesis (AMIC)
- Instant CEMTRO Cell (ICC), high density autologous chondrocytes implantation
- Regeneration of joint cartilage: perspectives and future
- Autologous chondrocyte implant surgery of the knee
- Full thickness chondral ulcers of the patella and femoral condyle of the knee
Más en PUBMED
Más en Google Scholar
Más en ORCID
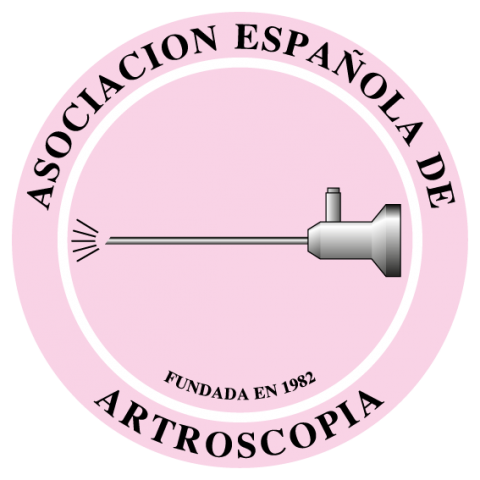

Revista Española de Artroscopia y Cirugía Articular está distribuida bajo una licencia de Creative Commons Reconocimiento-NoComercial-SinObraDerivada 4.0 Internacional.